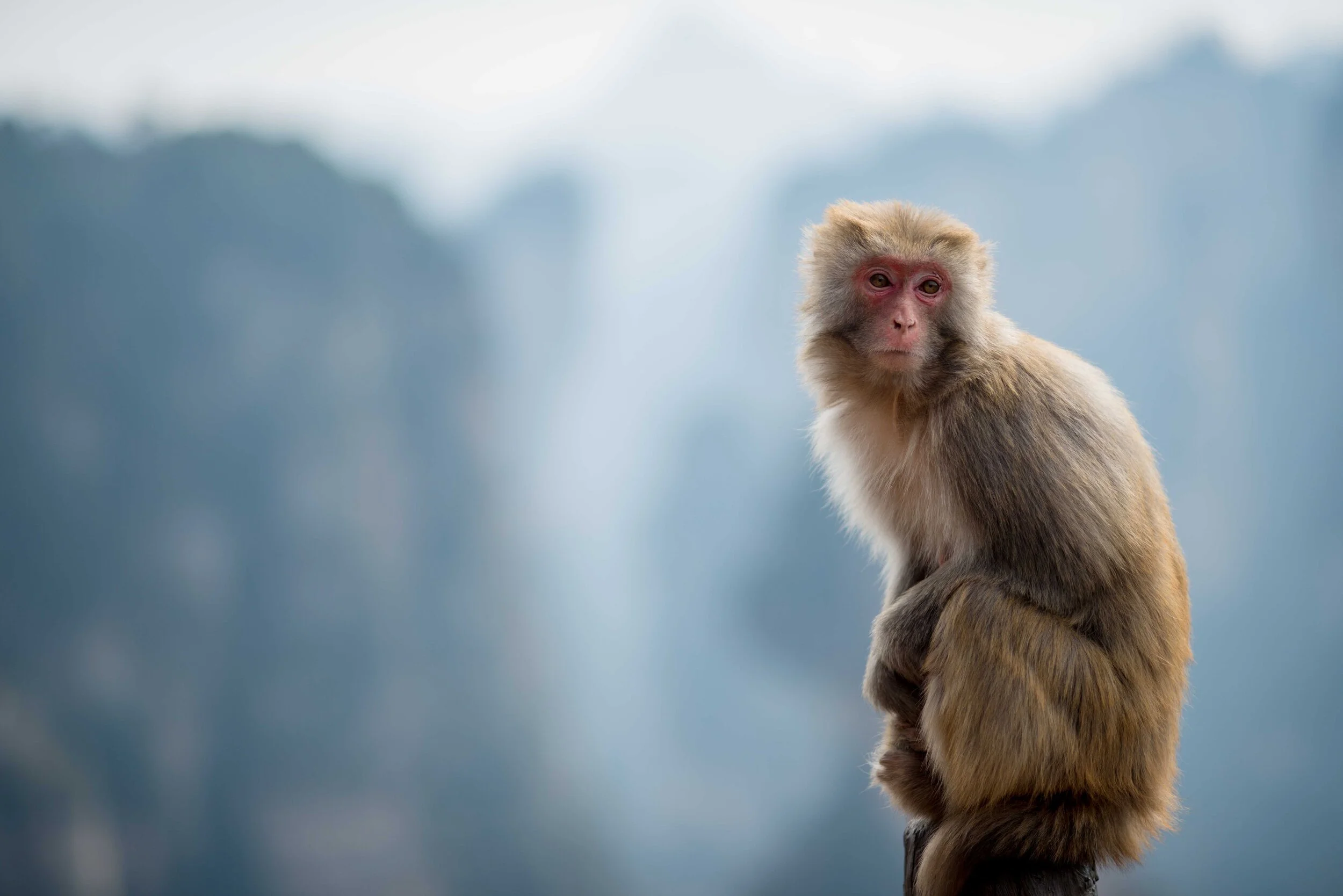
A Primate Model of an Intra-cortically Controlled FES Prosthesis for Grasp
Sandro Mussa-Ivaldi, Depts. of Physiology and Phys. Medicine and Rehab.
Northwestern University
Eric J. Perreault, Depts. of Biomedical Engineering and Phys. Medicine and Rehab
Northwestern University
Sara A. Solla, Depts. of Physiology and Physics & Astronomy
Northwestern University
People: Kevin Bodkin, Xuan Ma, Ege Altan, Fabio Rizzoglio
In the space of barely over ten years, Brain Computer Interfaces (BCIs) to restore movement have developed from the stuff of science fiction to clinically relevant devices. Signals recorded from the brain can be transformed into control signals that allow paralyzed humans to control the movement of a computer cursor or robotic limb voluntarily (Collinger et al. 2013; Weiss et al 2020). The algorithm used to transform the brain signals is referred to as a “decoder”. We have shown in previous studies that it is possible to use a BCI to control a Functional Electrical Stimulation (FES) system to restore voluntary movement even of a paralysed hand (Ethier et al., 2012). For those experiments we developed a unique model of spinal cord injury (SCI) using an implanted cannula system that delivers an anesthetic to cuffs placed around two key nerves in the arm of a monkey (Pohlmeyer et al., 2009). Injection of local anesthetic causes a nerve block that reproduces many of the acute effects of spinal cord injury, but lasts only one or two hours. Prior to the nerve block, we record signals from the brain and from muscles in the arm and hand (called electromyograms, or EMGs). We use the data to compute the decoders that transform the neural signals into predicted EMG signals. Some persons with paralyzed limbs already use neuroprosthetic devices based on FES (Peckham, Keith and Freehafer 1988; Peckham and Kilgore 2013; Lynch and Popovic 2008). The catch is that these systems must be controlled by the person’s limited remaining voluntary movements. That’s where BCIs come in. Following the onset of paralysis, our BCI uses the decoder to control FES based purely on signals from the monkey’s brain (Fig FES). Our monkeys could grasp objects voluntarily despite the temporary paralysis of their hand caused by the nerve block (see video here).
Fig FES: A monkey performs an isometric grasp task in the laboratory during temporary paralysis using a head-mounted wireless recording system and a wireless stimulation system located in a backpack. (A) Neural signals from the motor cortex, recorded while the monkey attempted to perform the task. (B) The neural signals are used to predict intended EMG (blue), which is then mapped onto time-varying FES commands (purple) that cause the muscles to contract. (C) Measured force (orange) modulates with the predicted EMG and FES commands. During catch trials (light blue boxes) the stimulator was turned off, demonstrating that the monkey was unable to produce force and perform the task without FES.
Existing BCIs, while remarkable, require the user to be wired to stationary equipment and the decoder to be frequently recalibrated. This limitation extends to our own previous efforts on BCI-controlled-FES. However, we are currently developing a wireless BCI that we anticipate will be able to operate 24 hours a day in the monkey’s home cage, restoring voluntary movement for a broad range of the monkey’s normal motor behaviors including foraging, feeding, and playing with toys. We use an implanted infusion pump to deliver anesthetic to the cuffs for indefinite periods of time, yet with full recovery of normal movements within a day of stopping the drug. While we have not yet implemented the full wireless FES system, this technology also gives us the ability to study the brain’s representation of movement across a range of motor behaviors that has never been possible before. We are currently studying the cortical and EMG signals produced by the monkey’s in-cage behaviors, and comparing them to the more typical signals collected in the lab.
Fig Lab vs. Unconstrained: Unlike in-lab tasks with the monkey’s body constrained in a primate chair and upper limbs moving in a constrained workspace, monkeys inside our telemetry enclosure perform various freeform movements unimanually or bimanually in the open workspace. We record cortical and EMG signals during these naturalistic behaviors like grooming, foraging, and climbing, as well as during the use of instrumented devices like those used on the lab.
Eventually, during the FES experiments, we will be able to study motor learning and adaptation without the limitations imposed by the intermittent availability of current BCIs. We will study the gradually changing brain activity as the monkeys learn to use this BCI-controlled-FES. This system will provide a platform close to that necessary for clinical translation, with which we will be able to study the limits of current decoders and to develop nonlinear and adaptive decoders designed to assist the monkey’s own adaptive processes. While this application is focused on restoration of grasp, its general principles will extend to the control of reaching, lower limb function, and even prosthetic limbs. Ultimately, this work will develop the interface, decoder, and control technology that will be necessary to move BCIs from the lab to the clinic, and home.
Fig Cage Signal: Typical M1 activity and EMG signals from wireless recordings during monkey’s different unconstrained behaviors inside the telemetry cage. Upper panel: M1 firing rates. Lower panel: rectified and low-pass filtered EMGs from flexor carpi ulnaris (FCU), flexor digitorum profundus (FDP), extensor carpi radialis (ECR), first dorsal interossei (fDI) and supinator (Sup).
Citations:
Collinger, J. L., Wodlinger, B., Downey, J. E., Wang, W., Tyler-Kabara, E. C., Weber, D. J., ... & Schwartz, A. B. (2013). High-performance neuroprosthetic control by an individual with tetraplegia. The Lancet, 381(9866), 557-564.
Jeffrey M. Weiss, Robert A. Gaunt, Robert Franklin, Michael L. Boninger & Jennifer L. Collinger (2020) Demonstration of a portable intracortical brain-computer interface, Brain-Computer Interfaces, DOI: 10.1080/2326263X.2019.1709260
Ethier, Christian, Emily R. Oby, Matthew J. Bauman, and Lee E. Miller. "Restoration of grasp following paralysis through brain-controlled stimulation of muscles." Nature 485, no. 7398 (2012): 368.
Pohlmeyer, E. A., L. R. Jordon, P. Kim and L. E. Miller (2009). "A fully implanted drug delivery system for peripheral nerve blocks in behaving animals." J Neurosci Methods 182: 165-172.
Peckham, P., M. Keith and A. Freehafer (1988). "Restoration of functional control by electrical stimulation in the upper extremity of the quadriplegic patient." The Journal of Bone and Joint Surgery 70(1): 144.
Peckham, P. H. and K. L. Kilgore (2013). "Challenges and Opportunities in Restoring Function After Paralysis." Biomedical Engineering, IEEE Transactions on 60(3): 602-609.
Lynch, C. L. and M. R. Popovic (2008). "Functional electrical stimulation." Control Systems, IEEE 28(2): 40-50.